Unlocking the Future of Immunization – The Rise of Systems Vaccinology in Modern Vaccine Development
In the ever-evolving landscape of immunology, the emergence of systems biology has catalyzed a transformative approach to vaccine development, known as systems vaccinology.
This interdisciplinary field transcends traditional methodologies by integrating vast amounts of biological data to provide a holistic understanding of how vaccines interact with the immune system. By examining the immune response as a complex, interconnected system, systems vaccinology offers unprecedented insights that promise to revolutionize vaccine development, improve efficacy, and pave the way for personalized medicine. As we delve into this groundbreaking approach, we explore its evolution, key components, and the future potential it holds for advancing global immunization efforts.
Introduction to Systems Vaccinology
In the rapidly evolving field of immunology, systems biology has paved the way for a revolutionary approach to vaccine development known as systems vaccinology.
Systems biology is an interdisciplinary approach to studying and understanding complex biological systems by examining them as integrated wholes rather than focusing solely on individual components. Systems biology takes a holistic view of biological systems, considering how different parts interact to produce emergent properties and behaviors that cannot be understood by studying components in isolation.1 This contrasts with the more traditional reductionist approach in biology.2 This approach allows researchers to model and predict how changes at the molecular level can impact the behavior of an entire biological system, providing deeper insights into health, disease, and the effects of therapeutic interventions. It integrates diverse types of biological data, including genomics, proteomics, metabolomics, and other “-omics” technologies. Systems biology combines experimental data with computational modeling and mathematical analysis to develop a comprehensive understanding of biological systems.3
Systems vaccinology applies systems biology principles to vaccine research and development. It aims to provide a comprehensive understanding of vaccine-induced immune responses and predict vaccine efficacy. Much like systems biology, it examines the entire immune response to vaccination, considering how different parts of the immune system interact to produce protective immunity.4 A key goal of systems vaccinology is to develop predictive models of vaccine efficacy. By analyzing early molecular signatures induced by vaccination, researchers aim to predict the later development of protective immune responses. Systems vaccinology can reveal novel mechanisms of immune regulation and identify early innate signatures that correlate with vaccine immunogenicity5.
By providing a more comprehensive understanding of vaccine-induced immunity, systems vaccinology has the potential to accelerate vaccine development, improve vaccine efficacy, and enhance our ability to protect against challenging pathogens and emerging infectious diseases.
The Evolution of Vaccine Development
From traditional empirical methods to rational design
The evolution of vaccine development has seen a remarkable transformation from traditional empirical methods to modern rational design approaches. This shift has been driven by advancements in technology, our growing understanding of immunology, and the need for more efficient vaccine development processes.
In the early days of vaccinology, vaccine development relied heavily on empirical methods. These traditional approaches involved:
- Attenuation: Weakening pathogens through repeated passages in animal or cell cultures.
- Inactivation: Killing pathogens using heat or chemicals while maintaining their immunogenic properties.
While these methods led to successful vaccines against diseases like smallpox and polio, they were time-consuming and often involved trial and error6.
The advent of genomic sequencing in the 1990s marked a turning point in vaccine development. The sequencing of the Haemophilus influenzae genome in 1995 paved the way for “Reverse Vaccinology,” a genome-based approach to vaccine development. This method allowed researchers to:
- Identify potential antigens directly from the pathogen’s genome.
- Predict immunogenic proteins without growing the pathogen in culture.
- Accelerate the discovery of novel vaccine candidates.
Today’s rational design approaches in vaccine development incorporate:
- Systems biology: Using multi-omics technologies to understand immune responses at a molecular level.
- Bioinformatics: Employing computational tools to analyze large datasets and predict vaccine efficacy and safety.
- Structural vaccinology: Designing antigens based on the three-dimensional structure of pathogen proteins.
These methods have led to significant achievements, such as the development of the four-component meningococcus B vaccine (4CMenB), which has shown 82.9% effectiveness in infants in the UK.
By embracing these rational design methods, vaccine development has become more targeted, efficient, and capable of addressing complex pathogens that were previously difficult to tackle using traditional approaches.
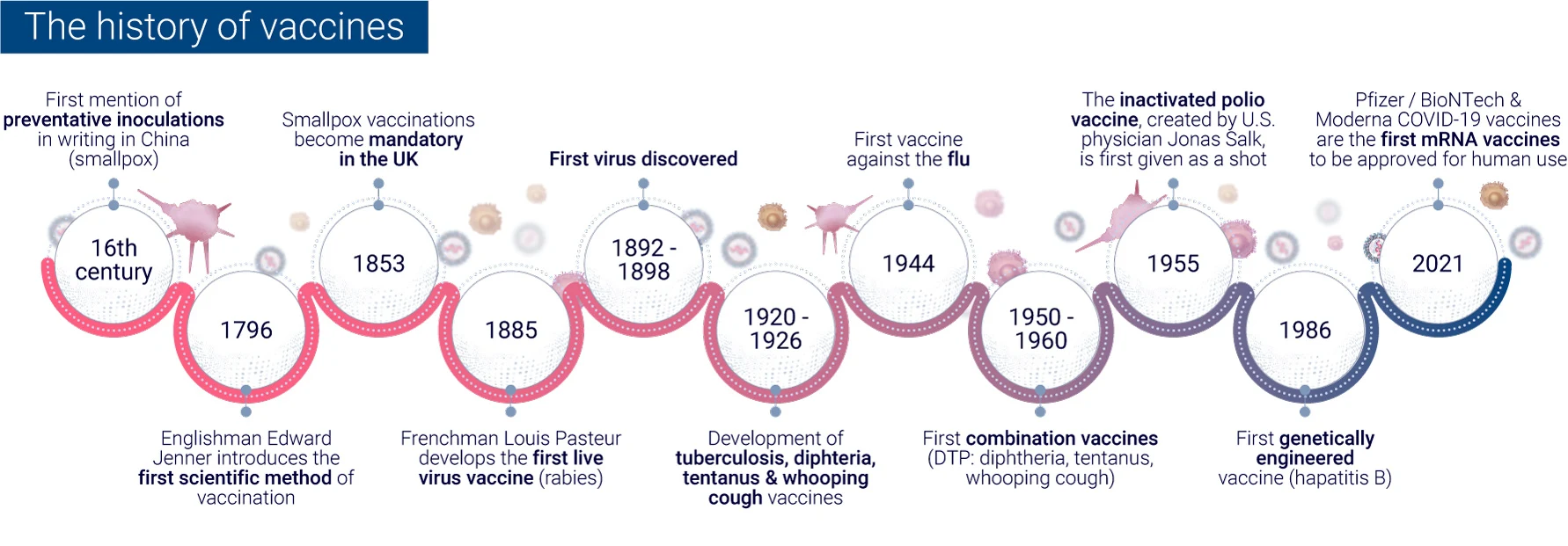
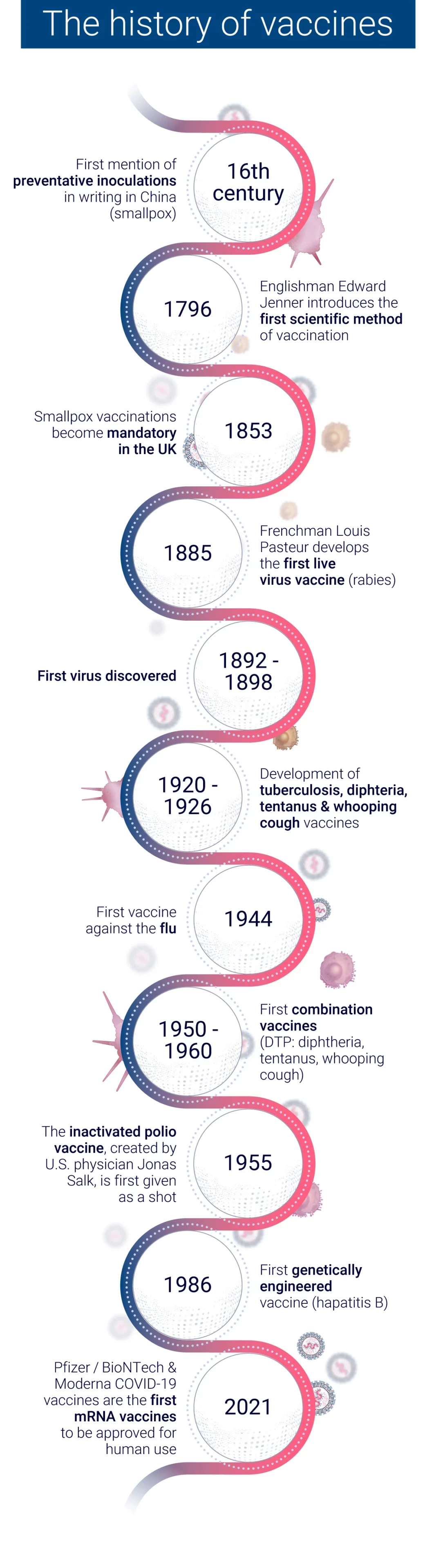
The role of big data and computational tools in identifying effective vaccine candidates
Building on the evolution of vaccine development, big data, and computational tools have revolutionized the process of identifying effective vaccine candidates. These advanced technologies have enabled researchers to:
- Analyze vast genomic and proteomic datasets to identify potential antigens across multiple pathogens simultaneously7.
- Utilize machine learning algorithms to predict the immunogenicity and efficacy of vaccine candidates, significantly reducing the time and resources required for initial screening.
- Employ high-throughput sequencing techniques to generate large volumes of biomedical data, which can be mined for suitable biological products and appropriate targets like genes and proteins.
The integration of big data analytics has also facilitated8:
- Virtual screening of hundreds of billions of compounds in minutes, dramatically accelerating the early stages of vaccine development.
- Analysis of gene expression signatures using data from repositories like The Cancer Genome Atlas (TCGA) and NCBI Gene Expression Omnibus (GEO) to explore new biomarkers and potential targets.
- Implementation of artificial intelligence models for tasks such as chemical compound identification, target validation, and vaccine efficiency evaluation.
Furthermore, big data approaches have enhanced our understanding of immune responses to vaccines. By analyzing large-scale datasets from clinical trials and post-marketing surveillance, researchers can identify early innate signatures that predict vaccine immunogenicity and uncover novel mechanisms of immune regulation.
These advancements have not only accelerated the vaccine development process but also improved its precision, allowing for more targeted and potentially more effective vaccine candidates to be identified and developed. The COVID-19 pandemic has further highlighted the critical role of these technologies in rapidly responding to emerging infectious threats9.
Key Components of Systems Vaccinology
Multi-omics technologies and how they contribute to understanding the immune response
In systems vaccinology, multi-omics technologies are crucial in providing a comprehensive understanding of the immune response to vaccines. These technologies allow researchers to analyze various biological molecules and their interactions at different levels, offering a holistic view of the immune system’s response. Here’s how different “omics” approaches contribute to this field.
Genomics focuses on analyzing the entire genetic makeup of an individual. In vaccine research, it helps identify genetic variations that may influence vaccine responses. Techniques such as single nucleotide polymorphism (SNP) analysis and whole-genome sequencing are employed to uncover genetic factors affecting immune responses10.
Transcriptomics examines gene expression patterns in response to vaccination. RNA sequencing (RNA-seq) is commonly used to identify genes and pathways activated by vaccines, predict vaccine efficacy based on early gene expression signatures, and understand host-pathogen interactions at the molecular level11.
Proteomics studies the entire set of proteins expressed within a cell or tissue. In vaccinology, it’s used to identify T cell and B cell epitopes, map antibody specificities, and analyze protein expression dynamics post-vaccination.
Mass spectrometry and protein arrays are key techniques that drive these insights.
Metabolomics explores the small molecule metabolites present in biological samples. In vaccine research, metabolomics has revealed metabolic signatures associated with vaccine efficacy and interactions between the metabolome and immune responses. It has also helped identify potential metabolic adjuvants that could enhance vaccine potency.
Epigenomics investigates gene expression changes that don’t involve alterations to the DNA sequence. In vaccinology, epigenomics helps understand how vaccines induce long-term immune memory, age-related differences in vaccine responses, and the influence of environmental factors on vaccine efficacy.
Systems serology integrates multiple assays to comprehensively characterize antibody responses. This includes analyzing antibody levels, isotypes, and subtypes, antibody functionality and glycosylation patterns, and Fc receptor binding properties.
By integrating data from these omics approaches, researchers can construct a more complete picture of the immune response to vaccines. This multifaceted perspective enables:
- The identification of molecular signatures that can predict vaccine efficacy.
- A deeper understanding of the mechanisms driving protective immunity.
- The development of more targeted and effective vaccine formulations.
- The personalization of vaccination strategies tailored to individual immune profiles.
The integration of these diverse datasets requires advanced computational tools and bioinformatics approaches, highlighting the interdisciplinary nature of systems vaccinology.
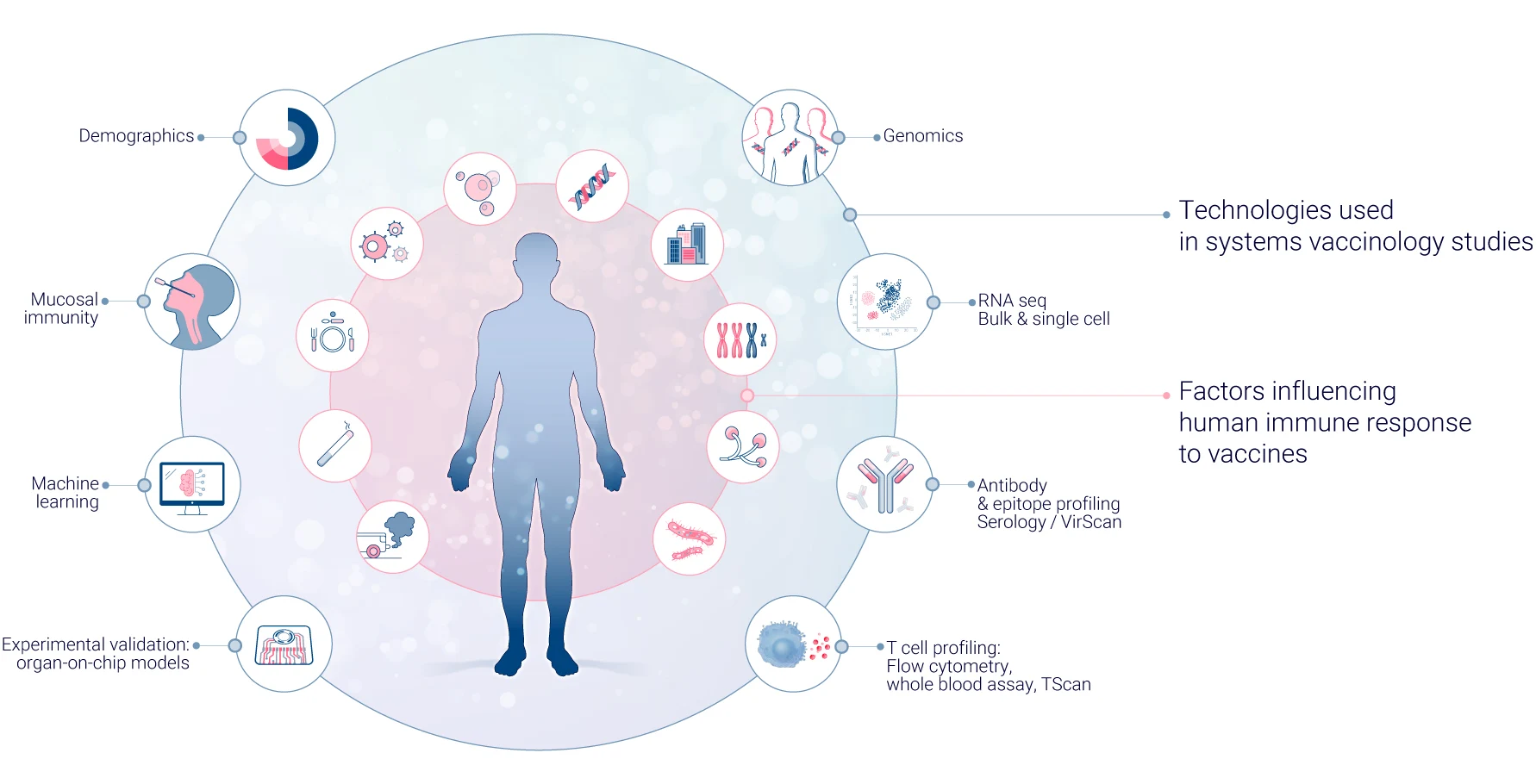
How systems vaccinology helps in predicting vaccine efficacy and immunogenicity
Systems vaccinology has advanced our ability to predict vaccine efficacy and immunogenicity, leveraging a suite of innovative approaches that bring unprecedented insights.
By integrating data across multiple “omics” technologies, researchers can identify more robust biomarkers that reflect the full complexity of vaccine responses. This multi-modal approach not only identifies comprehensive signatures but also reveals novel correlations across genomic, transcriptomic, and proteomic layers that would remain hidden in isolated analyses. Advanced computational methods, including machine learning and artificial intelligence, are applied to these large datasets, creating predictive models that forecast vaccine outcomes based on early immune signatures and identifying intricate patterns in immune responses beyond the reach of traditional statistics.
Moreover, network analysis in systems vaccinology maps the intricate interactions within the immune system, pinpointing key regulatory hubs and pathways that drive effective immunity while also predicting potential adverse reactions. The field is also moving towards personalized vaccine response predictions, tailoring formulations to individual genetic and immunological profiles, and optimizing vaccination schedules based on personalized immune dynamics. Temporal dynamics analysis provides insights into the kinetics of immune response development, allowing researchers to identify critical time points for assessing efficacy and predicting long-term immunity.
Cross-vaccine comparisons enabled by these systems approaches are shedding light on common mechanisms among successful vaccines, offering pathways to enhance the design and efficacy of new vaccines across different types. These advancements are pushing the boundaries of vaccine research, providing new tools and strategies to predict and enhance vaccine efficacy and immunogenicity, surpassing the capabilities of traditional methods.
Challenges and Future Directions
Challenges faced in systems vaccinology
Systems vaccinology, while promising, faces several significant challenges that researchers are actively working to overcome. One of the primary hurdles is the sheer complexity of integrating the vast amounts of data generated by multi-omics technologies.11 This includes the need to coherently combine heterogeneous data from genomics, transcriptomics, proteomics, and metabolomics, while also accounting for the temporal dynamics of immune responses and integrating information across molecular, cellular, and organismal scales.14 Additionally, the inherent biological variability of the immune system—driven by differences in genetic backgrounds, environmental factors, and microbiome compositions—compounds the complexity, leading to highly variable vaccine responses both between and within individuals over time.
From a computational perspective, the analysis of such big data also presents challenges. Developing robust statistical methods to manage high-dimensional data with relatively small sample sizes, adapting machine learning algorithms to vaccine-specific contexts, and achieving a balance between sensitivity and specificity in predictive models are ongoing areas of focus, particularly when translating systems-level insight into practical clinical vaccine development. The translational gap is another critical challenge, as is the evolving regulatory landscape for incorporating these insights into vaccine approval processes.
Standardization and reproducibility across studies and laboratories remain crucial yet difficult to achieve due to the complexity of experimental protocols and the variability of biological systems. Ethical and privacy concerns also come to the forefront, particularly regarding the use and security of sensitive biological data, including genomic information. Finally, the resource-intensive nature of systems vaccinology, with its high costs and the need for interdisciplinary expertise, poses a barrier to widespread adoption.4
Overcoming these challenges requires sustained collaboration among immunologists, bioinformaticians, statisticians, and clinicians. As the field advances, new methodologies and technologies are being developed to address these obstacles, paving the way for more effective vaccine design and evaluation.
Future potential
Systems vaccinology holds significant promise for the future of vaccine development and personalized medicine.
As these approaches are applied to larger and more diverse populations, we can anticipate the emergence of more robust and generalizable predictive models of vaccine efficacy. These models will be enriched by identifying population-specific immune signatures, enabling tailored vaccination strategies that consider factors such as age, genetics, and environmental influences.
The development of advanced immune-monitoring tools will further enhance our ability to profile immune responses with greater precision. Single-cell multi-omics approaches, for example, will offer unprecedented insights into cellular heterogeneity, while advances in proteomics and metabolomics will provide a more comprehensive understanding of immune system dynamics. Improved in situ imaging techniques may even allow real-time monitoring of immune responses, offering new opportunities for studying vaccine effects as they occur.
The application of artificial intelligence and machine learning to systems vaccinology data will likely lead to more accurate predictive models of vaccine efficacy and safety, uncover novel biomarkers, and accelerate vaccine design through in silico modeling. These technologies could also pave the way for truly personalized vaccination strategies, where vaccine formulations and schedules are tailored to an individual’s genetic and immunological profile, optimizing efficacy and safety.
Systems vaccinology is also poised to revolutionize our response to emerging diseases. By leveraging insights from existing vaccine data, researchers could develop vaccines against new pathogens more rapidly, monitor and predict vaccine efficacy in real-time during outbreaks, and identify at-risk populations requiring modified vaccination strategies. Furthermore, the identification of common mechanisms among successful vaccines may lead to the creation of universal vaccine platforms, which can be swiftly adapted to combat new or highly variable pathogens like influenza or HIV.
As the field progresses, increased integration with other research areas—such as microbiome studies, epigenetics, and immunometabolism—will be crucial for fully understanding how vaccines interact with the various systems in the human body and for optimizing their design. These advancements hold the promise of revolutionizing vaccine development, making it more efficient, effective, and personalized to meet both individual and population needs. However, achieving this potential will require ongoing technological innovation, interdisciplinary collaboration, and careful navigation of ethical and regulatory challenges.
Concluding Thoughts
As we stand on the brink of a new era in vaccine development, systems vaccinology offers a beacon of hope for overcoming the challenges of emerging infectious diseases and tailoring vaccines to individual needs. By harnessing the power of multi-omics technologies, advanced computational tools, and interdisciplinary collaboration, this approach is poised to accelerate vaccine discovery, enhance efficacy, and ensure safer, more personalized immunization strategies. However, realizing the full potential of systems vaccinology will require continued innovation, rigorous standardization, and a commitment to addressing ethical and regulatory complexities. As the field progresses, its impact on public health could be profound, unlocking new possibilities for preventing and controlling diseases in a rapidly changing world.
References
2Systems Biology as Defined by NIH
3What is systems biology? — BioSyL – Réseau de Biologie Systémique de l’Université de Lyon
4Pulendran B, Li S, Nakaya HI. Systems vaccinology. Immunity. 2010 Oct 29;33(4):516-29. doi: 10.1016/j.immuni.2010.10.006. PMID: 21029962; PMCID: PMC3001343.
5Zhu, H., Chelysheva, I., Pollard, A.J. et al. Spotlight on systems vaccinology: a novel approach to elucidate correlates of protection. Genes Immun 25, 336–337 (2024). https://doi.org/10.1038/s41435-023-00247-2
6Andreano E, D’Oro U, Rappuoli R and Finco O (2019) Vaccine Evolution and Its Application to Fight Modern Threats. Front. Immunol. 10:1722. doi: 10.3389/fimmu.2019.01722
7Bragazzi NL, Gianfredi V, Villarini M, Rosselli R, Nasr A, Hussein A, Martini M, Behzadifar M. Vaccines Meet Big Data: State-of-the-Art and Future Prospects. From the Classical 3Is (“Isolate-Inactivate-Inject”) Vaccinology 1.0 to Vaccinology 3.0, Vaccinomics, and Beyond: A Historical Overview. Front Public Health. 2018 Mar 5;6:62. doi: 10.3389/fpubh.2018.00062. PMID: 29556492; PMCID: PMC5845111.
8P. Zhao & X. Chen (2022). The Role of Big Data Analytics in Drug Discovery and Vaccine Development Against COVID-19. IGI Global.
9Aslam F, Yue Y. Big data analytics and COVID-19 vaccine. Comput Methods Programs Biomed Update. 2022;2:100062. doi: 10.1016/j.cmpbup.2022.100062. Epub 2022 Jun 28. PMID: 35783471; PMCID: PMC9236915.
10Wimmers F, Pulendran B. Emerging technologies for systems vaccinology – multi-omics integration and single-cell (epi)genomic profiling. Curr Opin Immunol. 2020 Aug;65:57-64. doi: 10.1016/j.coi.2020.05.001. Epub 2020 Jun 3. PMID: 32504952; PMCID: PMC7710534.
11Raeven RHM, van Riet E, Meiring HD, Metz B, Kersten GFA. Systems vaccinology and big data in the vaccine development chain. Immunology. 2019 Jan;156(1):33-46. doi: 10.1111/imm.13012. Epub 2018 Nov 13. PMID: 30317555; PMCID: PMC6283655.
12Kennedy RB, Ovsyannikova IG, Palese P, Poland GA. Current Challenges in Vaccinology. Front Immunol. 2020 Jun 25;11:1181. doi: 10.3389/fimmu.2020.01181. PMID: 32670279; PMCID: PMC7329983.
13Wimmers F, Pulendran B. Emerging technologies for systems vaccinology – multi-omics integration and single-cell (epi)genomic profiling. Curr Opin Immunol. 2020 Aug;65:57-64. doi: 10.1016/j.coi.2020.05.001. Epub 2020 Jun 3. PMID: 32504952; PMCID: PMC7710534.
14Nakaya HI, Pulendran B. Systems vaccinology: its promise and challenge for HIV vaccine development. Curr Opin HIV AIDS. 2012 Jan;7(1):24-31. doi: 10.1097/COH.0b013e32834dc37b. PMID: 22156839; PMCID: PMC3253345.
Comments are closed.