Understanding Pain & Neurogenic Inflammation
Can our understanding of pain and neurogenic inflammation help in predicting and managing injection-related pain?
Nociception, the neural processes of encoding and processing noxious stimuli, and the sensation of pain are initiated by extreme pressures, temperatures, toxic substances, and inflammatory mediators capable of causing tissue injury.
These strong stimuli are recognized by specific nerve cells in the body called nociceptors. Unlike the highly sensitive systems of sight, hearing, smell, taste, and touch that react to a wider range of stimuli, nociceptors are designed to respond only to conditions that could be harmful.1
The complex bidirectional communication between nociceptors, the body’s pain sentinels, and the immune system is crucial in shaping our perception of pain, particularly during subcutaneous injections. Here, we look into the fascinating science behind these interactions, exploring how nociceptors translate tissue damage into an “ouch” and how we could use that to predict pain upon injection.
What are sensory neurons?
Sensory neurons are specialized nerve cells that transmit sensory information from the periphery to the central nervous system. They are equipped with receptors and ion channels to detect stimuli like pressure, heat, and chemicals. Upon activation, they send electrical signals through peripheral nerves to the spinal cord and brain, where these signals are interpreted.
Sensory neurons can perform different functions depending on the type of stimuli and specific thresholds:
Mechanoreceptors act like tiny pressure gauges in our body which sense physical deformation like touch or pressure. Proprioceptors, dedicated to informing us about the position of our body parts, belong to this category.
Chemoreceptors can be categorized into two groups: (1) direct chemoreceptors, which interact with direct stimuli such as chemical substances (taste) or pH changes, and (2) distant chemoreceptors, which are stimulated by odor molecules.
Thermoreceptors are located in the skin and function as the body’s temperature gauges sensing heat and cold. When they sense a dramatic temperature shift, they trigger a rapid response, sending fast warning signals to your muscles to move you away from the danger.
Electromagnetic receptors, like photoreceptors in our eyes, detect energy such as light or electricity, allowing us to perceive the world around us.
Nociceptors are specifically designed to detect noxious or harmful stimuli. This process serves as a protective mechanism, alerting the organism to potential threats and prompting appropriate responses to avoid further injury.1
The sensory neurons innervate peripheral barrier tissues such as the skin, genitourinary tract, and the GI tract.
Nociception and pain perception
Noxious stimuli are potentially damaging signals, like extreme temperature and mechanical stimulation, provoke an avoidance response.
When a noxious stimulus is detected, ion channels are activated on the peripheral terminals of small, first-order primary afferent neurons, causing depolarization. This generates action potentials (a rapid sequence of changes in the voltage across a membrane) with frequencies proportional to the stimulus intensity, which travel along the axons of nociceptive Aδ and C fibers. These signals pass through the dorsal root ganglion (DRG) and reach the axon terminals in the spinal cord’s dorsal horn.
Aδ and C-fibers (figure 1) are both types of peripheral pain nerves that are classified by their diameter, function, and conduction velocity. Intense stimuli are relayed by medium-diameter, rapidly conducting Aδ fibers, which convey well-localized, fast “first pain.” In contrast, small-diameter, unmyelinated, slow-conducting C fibers transmit poorly localized, slow “second pain.”3
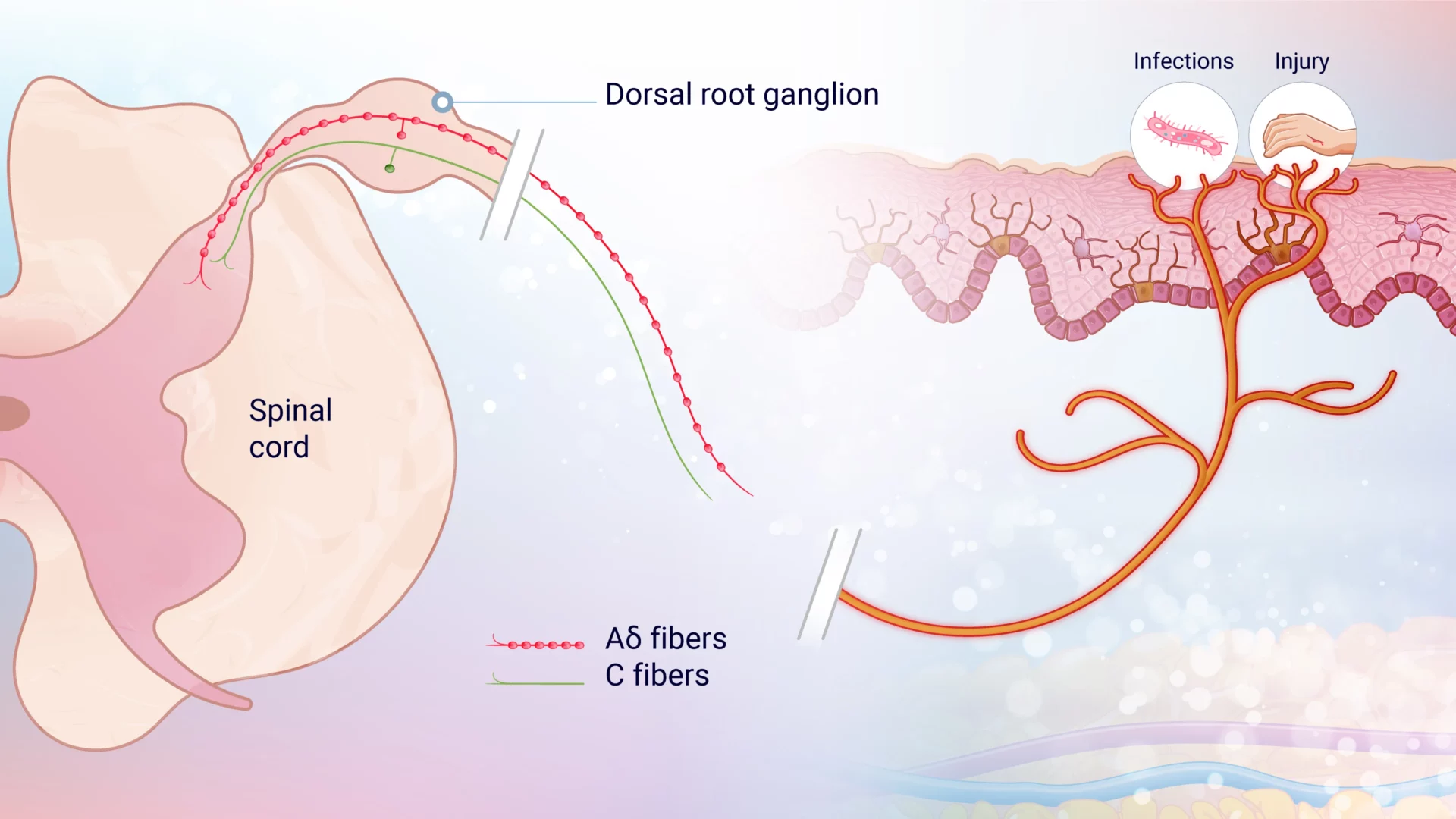
Pain manifests in various forms and intensities depending on the type and location of the stimulus. “First pain” is often described as sharp, stabbing, or pricking, while “second pain” is more widespread, characterized by burning, throbbing, cramping, and aching sensations, often accompanied by emotional distress described as “sickening.” These intense reactions highlight the importance of avoiding harmful situations to ensure survival and maintain homeostasis.3
Pain perception is highly individual and subjective, differing from the more objective nature of other senses. Factors such as stress can diminish the sensation of pain, while anticipation can amplify it, demonstrating the complex interplay between nociception and pain perception.
Interaction between immune system and nociceptors
The immune and nervous systems traditionally serve distinct roles: the immune system combats infections, maintains self-tolerance, and repairs tissue damage, while the nervous system transduces signals into electrical activity, leading to sensations and reflexes. However, the somatosensory nervous system is uniquely positioned to detect danger due to its dense network of nociceptors in tissues exposed to the external environment, such as the skin, lungs, urinary, and digestive tracts. Nociceptors, which are high-threshold pain-producing sensory fibers, can transduce noxious stimuli almost instantaneously. This rapid response makes the nervous system a potential “first responder” in host defense, acting much faster than the mobilization of the innate immune system.4
Nociceptives endings are found in the surroundings of keratinocytes, mast cells, and langerhans cells, and have the capacity to monitor the status of the skin.
When releasing neuronal factors (e.g., neuropeptides), nociceptive sensory neurons (i.e., nociceptors) play an important role in modulating the immune response. When noxious stimuli activate these sensory neurons, nociceptive signals are conducted from the peripheral axon toward the spinal cord and brain, which then triggers an appropriate behavioral response and the release of neuropeptides at the site of injury. These neuropeptides have direct immunomodulatory effects:
- Chemotaxis and Activation: They can attract and activate neutrophils, macrophages, and lymphocytes to the injury site and activate MRGPRX2+ mast cells to release their granular content.
- Vascular Effects: They can modulate the activation of vascular endothelial cells to increase blood flow, causing vascular leakage and promoting edema, which facilitates the recruitment of inflammatory leukocytes.
- Immune Cell Priming: Nociceptors can also prime dendritic cells to promote the differentiation of T helper cells into Th2 or Th17 subtypes. While these T cell subsets are typically involved in protecting the host from pathogens, they can also play a role in allergic reactions and autoimmune diseases by eliciting an inflammatory response.4
Neurogenic inflammation vs. Neuroinflammation?
Neurogenic inflammation refers to the spread of inflammation initiated by nociceptors (pain-sensing neurons) beyond the original site of activation. This process involves the propagation of inflammatory signals from nociceptors to surrounding cells, leading to the sensitization of nearby nociceptors.
Pro-inflammatory molecules released by activated nociceptors play a crucial role in this process. These molecules not only activate local inflammatory cells but can also directly stimulate other nociceptive nerve endings. Since most nociceptive nerve endings have receptors for these proinflammatory markers, the molecules can bind to and activate neighboring neurons that were not initially affected by the original stimulus. This cascade effect amplifies and sustains a neuroinflammatory response, contributing to the persistence and spread of pain and inflammation.5
Inflammatory mediators of pain
During inflammation, tissue-resident immune cells release mediators such as cytokines, lipids, proteases, and growth factors. These mediators bind to their receptors found on nociceptors. By doing so, they trigger signaling cascades that lead to phosphorylation and gating of ion channels which modify properties of nociceptor neurons. As a result, the action potential increases and ultimately, the threshold of sensitization decreases, leading to an increased responsiveness of the nociceptors.
Mast cells play a key part in the process. Upon activation, they degranulate and release cytokines (IL-5, TNFɑ, Il-6, IL-1β), 5HT (serotonin), histamine, and nerve growth factor (NGF), which results in pain sensitization through receptors on nociceptors (Figure 2).
Macrophages, monocytes, and T-cells play a part in the process of chronic pain.6
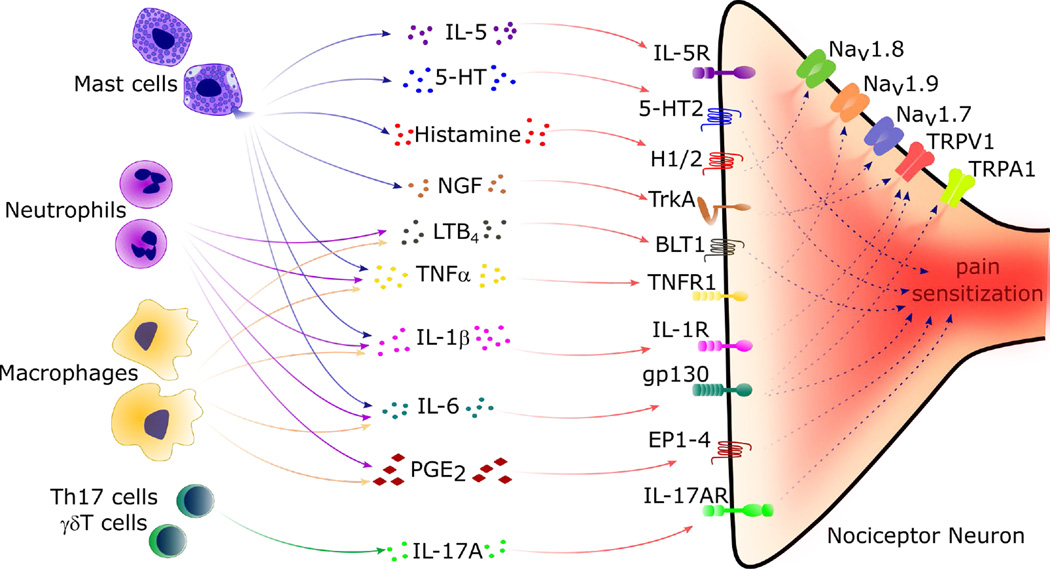
Mast cells & pain
Mast cells (MCs) are pivotal players in the inflammatory process, particularly in neurogenic inflammation. Situated in close proximity to peripheral sensory nerve endings, they act as one of the first responders when sensory nerves are activated. Upon activation by neuropeptides such as substance P or calcitonin gene-related peptide (CGRP), MCs release a variety of pro-inflammatory mediators, including cytokines and chemokines. This release can not only initiate but can also amplify the inflammatory response by recruiting other immune cells to the site of injury or infection.
In the skin, which is the largest organ that constantly interacts with the environment, neurogenic inflammation results from the activation of nerve endings and their secreted mediators. Sensory neurons expressing TRPV1 ion channels play a crucial role in this process. Activation of these sensory nerves triggers the release of neuropeptides, which can stimulate mast cells (MCs) to release additional inflammatory mediators.7 This intricate interaction between sensory nerve endings and MCs underpins the development and maintenance of cutaneous neurogenic inflammation (CNI), observed in conditions such as psoriasis, atopic dermatitis, prurigo, and rosacea. Activation of TRPV1 ion channels and the human receptor MRGPRX2 by substance P enhances the release of cytokines and chemokines, facilitating a robust inflammatory response.8 This highlights the significant role of MCs in bridging the nervous and immune systems, emphasizing their importance in neurogenic inflammation and their potential as therapeutic targets for treating inflammation-mediated pain.
Concluding remarks or how current knowledge on pain and neuroinflammation could help us predict pain following injection
Pain is a classic symptom of inflammation that involves nociceptors and the immune system. Pro-inflammatory mediators released locally following tissue damage or other stimuli lead to sensitization of the sensory nervous system both directly, by binding to their specific receptors present on peripheral nociceptor neurons, and indirectly, through a signaling cascade that influences action potentials.
While murine models have been widely used to elucidate the mechanism behind pain sensation and sensitization as well as the intricate dialogue between the sensory nervous system and the immune cells, their relevance can be discussed from a biological and immunological perspective.
Over the past decades, new analytical techniques have been developed and improved along with New Approach Methodologies (NAMs). These advancements have enabled us to assess the impact of chemical and therapeutics compounds dedicated for treating humans.
Today, the combination of live human ex vivo skin platforms, like the biostabilized and immunocompetent models from Genoskin, alongside advanced analysis techniques such as multiplex cytokine analysis unveils the intricacies of the skin’s immune response, while proteomics and transcriptomics provide a deep understanding of protein and gene expression. This synergy allows researchers to not only better understand the mechanism of action of injectable therapeutics, but also compare and refine formulations for optimal efficacy and patient comfort. Ultimately, this paves the way for treatments that better address patient needs and improve adherence, leading to a brighter future for human health.
Sources
1Dubin AE, Patapoutian A. Nociceptors: the sensors of the pain pathway. J Clin Invest. 2010 Nov;120(11):3760-72. doi: 10.1172/JCI42843. Epub 2010 Nov 1. PMID: 21041958; PMCID: PMC2964977.
2Chap 13.1. Oregon State University – Anatomy and Physiology. https://open.oregonstate.education/aandp/chapter/13-1-sensory-receptors/
3Woller SA, Eddinger KA, Corr M, Yaksh TL. An overview of pathways encoding nociception. Clin Exp Rheumatol. 2017 Sep-Oct;35 Suppl 107(5):40-46. Epub 2017 Sep 29. PMID: 28967373; PMCID: PMC6636838.
4Chiu IM, von Hehn CA, Woolf CJ. Neurogenic inflammation and the peripheral nervous system in host defense and immunopathology. Nat Neurosci. 2012 Jul 26;15(8):1063-7. doi: 10.1038/nn.3144. PMID: 22837035; PMCID: PMC3520068.
5Scott A.Armstrong, Michael J. Herr. Physiology, Nociception. Statpearls, NIH (https://www.ncbi.nlm.nih.gov/books/NBK551562/)
6Pinho-Ribeiro et al. Nociceptor Sensory Neuron–Immune Interactions in Pain and Inflammation, Trends in Immunology, January 20217, Vol.38, N˙1. http://dx.doi.org/10.1016/j.it.2016.10.001
7Marek-Jozefowicz, L.; Nedoszytko, B.; Grochocka, M.; Żmijewski, M.A.; Czajkowski, R.; Cubała, W.J.; Slominski, A.T. Molecular Mechanisms of Neurogenic Inflammation of the Skin. Int. J. Mol. Sci. 2023, 24, 5001. https://doi.org/10.3390/ijms24055001
8Green, D. P., Limjunyawong, N., Gour, N., Pundir, P., & Dong, X. (2019). A Mast-Cell-Specific Receptor Mediates Neurogenic Inflammation and Pain. Neuron, 101(3), 412–420.e3. https://doi.org/10.1016/j.neuron.2019.01.012
Comments are closed.