Navigating Unwanted Immunogenicity
Part 3 – Challenges & insights in biologic drug development
Over the past two and a half decades, biologics development has been growing exponentially, matching the pace of small molecules development in 20221.
Biologics, or biopharmaceutical products, encompass a wide range of therapeutic products such as vaccines, monoclonal antibodies, gene and cell therapy, and recombinant and fusion therapeutic proteins. Unlike small molecules that are chemically synthesized with a known structure, biologics usually have a quite complex configuration.2 In addition, biologics are produced in living organisms. Thus, due to their inherent nature, all biologics have an immunogenic potential.3
In the first blog article of our immunogenicity series, we explored the nuanced world of wanted versus unwanted immunogenicity, a pivotal aspect often encountered in vaccine development. This phenomenon, essentially the body’s adverse reaction to certain drugs, raises significant challenges in ensuring patient safety and efficacy of treatments. It underscores the importance of understanding the complex interactions between pharmaceuticals and the human immune system to mitigate potential risks. Our discussion extends beyond the basics, inviting readers to uncover a deeper understanding of the mechanisms and implications of drug-induced immunogenic responses.
Understanding unwanted immunogenicity
Immunogenicity is the propensity of a therapeutic compound to induce an immune response toward itself or endogenous proteins. In vaccine development, inducing an immune reaction is essential in establishing immunological memory and to protect against specific pathogens. However, it can be a significant drawback when developing therapeutic biologics.
Unwanted immunogenicity, also referred to as off-target immunogenicity, is the capacity of a compound to elicit an immune response directed against a therapeutic agent.
The immune response can result in various clinical outcomes, some benign while some other can be fatal. Off-target immunogenicity can lead to anti-drug antibody (ADAs) formation, which can lead to a diminution of drug efficacy. It can also result in hypersensitivity or allergic reactions.4
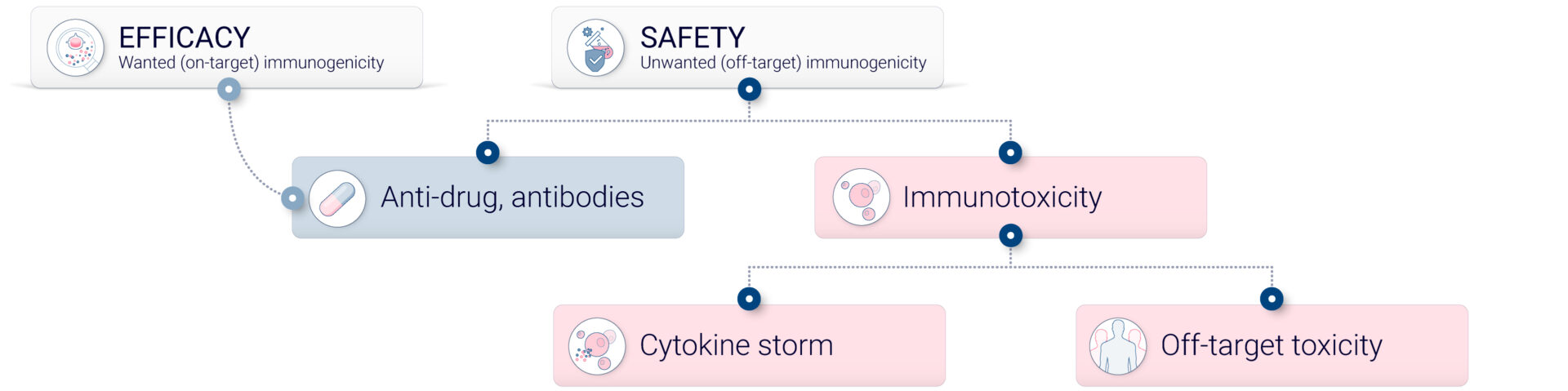
At the heart of it all is the immune system. The innate immune system, our primary line of defense, is triggered regardless of whether a therapeutic is given as a single dose or in multiple doses. The type of immune cells involved after the first dose is crucial in developing an adaptive immune response, including ADAs and allergic reactions. The characteristics of the biologic, combined with the patient’s inherent sensitivity, can lead to a range of unpredictable symptoms. This variability significantly contributes to the high attrition rate in drug development and the subsequent withdrawal of drugs from the market.
Different type of immunogenicity
Off-target immunogenicity can be categorized into several types, each with distinct characteristics and implications for patient safety and drug efficacy.
- Anti-drug antibodies
- Cross-reactive immunogenicity
- Neutralizing antibodies
- Cell mediated immunogenicity
- Protein aggregates
One of the most common forms of immunogenicity is the generation of ADAs. These are host antibodies directed against the therapeutic drug, which can neutralize the drug’s activity or alter its pharmacokinetics, leading to reduced drug efficacy and potentially hypersensitivity reactions. Cross-reactive immunogenicity occurs when the immune response generated against a therapeutic drug cross-reacts with a naturally occurring protein in the body, potentially leading to an autoimmune reaction or other adverse immune reaction. Neutralizing Antibodies (NAbs), a subset of ADAs, specifically neutralize the biological activity of the drug. For instance, in enzyme replacement therapies, NAbs can bind to and neutralize the therapeutic enzyme, leading to loss of efficacy.
Cell-mediated immunogenicity, where some drugs elicit a T cell-mediated immune response, can lead to delayed-type hypersensitivity reactions and is a critical consideration in drug safety. Additionally, therapeutic proteins can aggregate under certain conditions, enhancing the immune response; such protein aggregates are more immunogenic and can lead to increased ADA production. Furthermore, some small molecule drugs can act as haptens, binding to larger proteins and form adducts that are immunogenic, which can lead to drug-induced hypersensitivity reactions.
Factors contributing to unwanted immunogenic responses
The factors contributing to off-target immunogenicity can be categorized into three types:
- Patient-related factors such as genetic background or lifestyle habits
- Product-related factors such as inherent structural factor or impurities
- Treatment-related factors
Patient related factors
The patient’s inherent immune sensitivity and genetic background significantly influence the occurrence of unwanted immunogenicity, factors over which researchers have little or no control. However, the structure and nature of the therapeutic, as well as its formulation and route of administration, are adjustable aspects that can be optimized to mitigate off-target reactions.
Product-related factors
Product-related factors can have two different origins. They either are the results of the product nature or, they can be the consequence of the manufacturing process.
Recombinant native peptides and proteins
Even though recombinant proteins might share the same amino acid sequence as native proteins, the human immune system can still recognize them as foreign, potentially triggering an immune response. This immunogenicity doesn’t always cause issues but can lead to significant safety concerns, including:
- Reduced efficacy of the therapeutic protein due to antibody-mediated neutralization or removal
- Possible toxic reactions from the accumulation of antigen-antibody complexes
- Diminished levels of the naturally occurring version of the protein in the body, caused by the same antibody mechanisms targeting the native molecule.
Fusion proteins, which combine small peptides or hormones with larger carrier molecules like albumin, are studied for their ability to extend the therapeutic presence in the bloodstream. While they offer this advantage, they also raise concerns about potentially introducing new antigens at the junction of the fused components, the actual risk of which requires further empirical evaluation.5
Therapeutic antibodies
Monoclonal antibodies (mAbs) are powerful treatments targeting specific immune system markers to manage various immunopathological conditions. However, their high specificity also raises significant concerns about immunotoxicity, including potential adverse effects like immunosuppression, immunostimulation, and hypersensitivity. Despite the critical need for safety testing, non-clinical studies often fall short due to the lack of relevant animal models, particularly as many mAbs require testing in non-human primates (NHPs) to approximate human responses. Additionally, clinical trials frequently lack validated endpoints that could predict immunotoxicity effectively. This underscores the complexity and urgency of developing more predictive preclinical testing methods to ensure mAbs are safe before reaching clinical trials, given their unpredictable effects and novel mechanisms of action in targeting new biological pathways.6,7
The particular case of cytokine release-associated acute reactions.
Cytokine release, which became a major concern following a cytokine storm incident during a clinical trial in 2006, involves acute reactions ranging from mild flu-like symptoms to severe cytokine release syndrome and potentially multi-organ failure. The underlying mechanism of these reactions involves the direct activation of immunocompetent cells such as macrophages and lymphocytes by certain compounds, leading to the release of pro-inflammatory cytokines including IL-1β, TNFα, IFNβ, IFNγ, IL-6 and IL-8, which can vary in severity depending on the compound’s properties and the cells affected.7
The exact mechanisms behind acute infusion reactions from mAbs are not entirely clear. While cytokine release plays a significant role, the distinct clinical features observed in mAb infusion reactions, as opposed to typical flu-like symptoms seen with immunomodulatory cytokines, suggest additional processes may be involved, such as complement cascade activation seen in reactions to drugs like rituximab. Moreover, while antigen-specific (IgE-mediated) hypersensitivity is generally not expected after the first dose of a mAb—due to lack of prior sensitization—identifying such reactions becomes more complex with subsequent doses.7
Vaccines
Vaccines commonly produce mild adverse effects like inflammation, pain at the injection site, malaise, and slight fever, which are necessary to activate the immune system—a critical process for developing protective immunity. These effects reflect the activation of various immune components, which varies depending on the specific vaccine antigen and/or adjuvant used. Despite the minimal quantity of material administered, direct toxicity from vaccines is extremely rare. Most vaccine-related toxicities stem from the inflammatory response essential to the immune activation. Additionally, vaccines may contain excipients and preservatives, like antibiotics, which are included to prevent bacterial growth or are residues from the manufacturing process, and these too can contribute to observed toxicities.9
Impurities in vaccine manufacturing can significantly impact vaccine safety and efficacy, as illustrated by the comparison between ChAdOx1 nCoV-19 and Ad26.COV2.S vaccines. While the Ad26.COV2.S vaccine maintained low levels of impurities, analyses revealed that ChAdOx1 nCoV-19 exceeded regulatory limits for host cell proteins and free viral proteins, with some batches containing impurities 25 times above the European Medicines Agency’s specifications. This case underscores the critical need for stringent purification processes and tailored quality assays in the production of vectored vaccines to ensure their safety and effectiveness.10
Cell & Gene therapies
Cell and gene therapies (GTs) represent revolutionary advancements in treating serious diseases but are accompanied by significant safety challenges. The immunogenicity of recombinant adeno-associated virus (rAAV)-based GTs, as an example, poses a critical safety issue, as both the viral vector components and the therapeutic genes themselves can trigger immune responses. These responses can range from mild to severe, with pre-existing immunity to AAV vectors or newly induced immune reactions potentially reducing treatment efficacy or leading to severe adverse events. Despite these challenges, recent successful approvals of rAAV-based therapies highlight their potential, although the broad application remains limited by variable levels of natural immunity in different populations.11
Here again, the risks of immunogenicity of rAAV gene therapy can be influenced by a variety of factors including the product itself, the manufacturing process, treatment protocols, and patient-specific characteristics. Critical to both the efficacy and safety of these therapies are several types of immune responses that must be carefully managed. These include:
- the innate immune response
- pre-existing and treatment-induced or -boosted anti-AAV antibodies
- anti-transgene protein antibodies
- cellular responses, particularly T cell responses to AAV or transgene protein peptides presented on the surface of transduced cells.
These immune responses can significantly impact the therapeutic outcome, necessitating thorough consideration in the development and application of GTs.10
Treatment-related factors
The subcutaneous (SC) route of administration for biologics offers distinct advantages such as enabling patient self-administration and reducing healthcare costs associated with hospital-administered treatments. However, this route also presents unique immunogenicity challenges compared to intravenous (IV) administration. These challenges arise from the first-pass interactions of the therapeutic proteins with resident immune cells at the SC injection site, notably skin-resident dendritic cells and monocyte-derived dendritic cells. Such interactions, along with the antigen presentation mechanisms in the skin, contribute to a potentially heightened immune response. Furthermore, the SC route facilitates frequent encounters with dynamic skin antigen-presenting cells, which can lead to a more efficient and effective host immune response, offering both benefits and complexities in vaccine and biologic therapy development.
Concluding remarks
With the complex and crucial topic of unwanted immunogenicity in biologics, we have explored various types of immunogenic responses, such as the generation of ADAs, hypersensitivity reactions, and T cell-mediated responses, which can significantly affect patient safety and drug efficacy. Key factors influencing these responses include patient genetics, the inherent properties of the biologic, and the route of administration, with each playing a role in the potential immunogenic risks associated with therapy.
As the biotech and pharmaceutical industries continue to advance and bring new therapies to market, it is imperative to focus on improving predictive assays and refining therapeutic design to mitigate these risks. The insights provided in this series underscore the importance of ongoing research and collaboration across the industry. By enhancing our understanding of immunogenicity and its mechanisms, we can develop safer, more effective biologic therapies. The journey toward better patient outcomes and reduced immunogenicity is ongoing, and it is through sustained innovation and cooperative efforts that significant progress will be made.
Sources
1Senior, M. Fresh from the biotech pipeline: fewer approvals, but biologics gain share. Nat Biotechnol 41, 174–182 (2023). https://doi.org/10.1038/s41587-022-01630-6
3Garcês, Sandra, and Jocelyne Demengeot. “The Immunogenicity of Biologic Therapies.” Current problems in dermatology vol. 53 (2018): 37-48.
4Wadhwa, Meenu, and Robin Thorpe. “Unwanted immunogenicity: lessons learned and future challenges.” Bioanalysis vol. 2,6 (2010): 1073-84.
5Wendy Halpern, David Hutto,Chapter 25 – Biopharmaceuticals,Editor(s): Wanda M. Haschek, Colin G. Rousseaux, Matthew A. Wallig,Haschek and Rousseaux’s Handbook of Toxicologic Pathology (Third Edition),Academic Press,2013,Pages 751-782,ISBN 9780124157590
6Brennan, F. R., Cavagnaro, J., McKeever, K., Ryan, P. C., Schutten, M. M., Vahle, J., Black, L. E. (2018). Safety testing of monoclonal antibodies in non-human primates: Case studies highlighting their impact on human risk assessment. mAbs, 10(1), 1–17
7Descotes J. Immunotoxicity of monoclonal antibodies. MAbs. 2009 Mar-Apr;1(2):104-11. doi: 10.4161/mabs.1.2.7909. Epub 2009 Mar 19. PMID: 20061816; PMCID: PMC2725414
8Suntharalingam G, Perry MR, Ward S, Brett SJ, Castello-Cortes A, Brunner MD, et al. Cytokine storm in a phase 1 trial of the anti-CD28 monoclonal antibody TGN1412. N Engl J Med. 2006;355:1018–1028.
9Green MD, Hussain Al-Humadi N. Preclinical Toxicology of Vaccines. A Comprehensive Guide to Toxicology in Preclinical Drug Development. 2013:619–45
10Krutzke L, Rösler R, Allmendinger E, Engler T, Wiese S, Kochanek S. Process- and product-related impurities in the ChAdOx1 nCov-19 vaccine. Elife. 2022 Jul 4;11:e78513.
11Yang TY, Braun M, Lembke W, McBlane F, Kamerud J, DeWall S, Tarcsa E, Fang X, Hofer L, Kavita U, Upreti VV, Gupta S, Loo L, Johnson AJ, Chandode RK, Stubenrauch KG, Vinzing M, Xia CQ, Jawa V. Immunogenicity assessment of AAV-based gene therapies: An IQ consortium industry white paper. Mol Ther Methods Clin Dev. 2022 Aug 2;26:471-494.
Comments are closed.